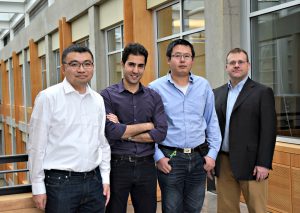
Graduate students Tong Zhang, Ali Najafi, Chenxin Su and Associate Professor Chris Rudell.
Nearly all commercial mobile transceivers (cellular phones, WiFi, Bluetooth, etc.) operate in the radio frequency band (1-to-5 GHz [gigahertz]). Due to favorable properties such as the required size of hardware and radio signal propagation loss, the 1-to-5 GHz band is considered the “beachfront real estate” of spectrum allocation. One gigahertz is equal to 1 billion hertz, or one cycle rotation of frequency. Because of this, many U.S. cellular phone carriers pay billions of dollars to acquire a small frequency band of a few megahertz (1 million hertz).
Although the commercial bands are completely occupied by communication applications, including emergency services, Wi-Fi, cellular networks, GPS and Bluetooth devices (as shown in Figure 1), the demand for higher data rates (driven mainly by video) continues with some estimating an increase by as much as 10 times in the next five years.
Electrical engineers in the Future Analog System Technologies (FAST) Lab at the University of Washington, recently demonstrated a novel radio system that uses a new high-frequency integrated CMOS chip and embedded processor. The technique, known as Full Duplex Communication, is capable of operating a single radio’s transmitter and receiver simultaneously, using a single carrier frequency on the same channel (as shown in Figure 2). This allows for better use of the existing commercial radio spectrum.
The UW EE researchers have been investigating Full Duplex Communication for the last five years. The research, which was reported at the 2017 IEEE International Solid-State Circuits Conference (ISSCC) and in a recent paper, set a new world record with respect to linearity, bandwidth and transmitter power output when the radio is used in the full duplex mode. This would allow the radio to operate in full duplex mode over significantly longer distances as compared to prior state-of-the-art. For example, this radio is the first to demonstrate performance potentially compatible with future 5th Generation (5G) wireless standards.
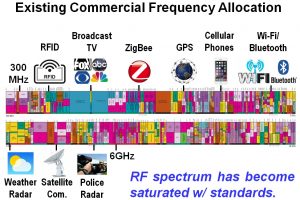
Figure 1: Federal Communication Commission (FCC) Existing Frequency Allocation in the RF and Sub-Millimeter Wave Bands
“Full Duplex Communication has the potential to double the efficiency of spectral use,” said lead author and electrical engineering graduate student Tong Zhang. “It does this by combining the traditional transmit and receive bands into one common frequency channel. This works for any wireless application with two communication, including cellular, Wi-Fi and Bluetooth radios.”
Currently, all radios reduce the possible interaction between a single user’s transmitter (TX) and receiver (RX) by either performing Frequency Domain Duplexing (FDD), which allows a single user to simultaneously transmit on one frequency while receiving a signal on another frequency, or by communicating using Time Division Multiplexing (TDD), where both the transmitter and receiver operate at different times, using different assigned frequencies. Both techniques are used to minimize interference from a given radio’s transmitter to the receiver. By way of example, Verizon phones use an FDD-based system to communicate, while AT&T phones often use TDD. With both FDD and TDD radios, a single user occupies two frequency bands to wirelessly communicate with another radio or base station. In contrast, Full Duplex Communication (as shown in Figure 2) allows a single user’s radio to simultaneously transmit and receive using the same frequency band, thus cutting in half the required frequency allocation of a single transceiver (e.g. a cell phone).
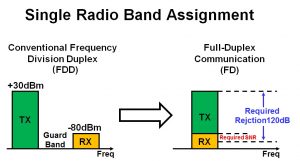
Figure 2: Channel Allocation for a Single User (radio) for the case of both traditional (existing) Frequency Division Duplex (FDD) and Future Full-Duplex (FD) Systems.
“This is analogous to a single lane supporting vehicle traffic in both directions, effectively reducing the needed real estate by half,” said lead faculty and electrical engineering associate professor Chris Rudell. “The obvious challenge of using a single-lane road to support two-way traffic is the potential collision between cars moving in opposite directions. If one radio were to transmit and receive a signal using the same assigned carrier frequency, this leads to a similarly challenging problem of the transmitter presenting interference to its own radio receiver.”
A typical cellular radio may transmit up to 1 Watt of power while very far from the base station, implying that the received signal is very small (as little as a few microvolts [one millionth of a volt] at the antenna). This is shown in Figure 2. Therefore, full duplex radios must supply more than 120 decibels of transmitter self-interference cancellation before the receiver can properly function.
Detecting a weak received radio signal, in the presence of a transmitter blasting at maximum output power, is equivalent to hearing someone whisper from the opposite side of the stadium, over the roar of the crowd, just after a Seahawks touchdown. This demands that the analog, baseband and digital components of the radio achieve sufficient cancellation of the transmitted signal inside the receiver.
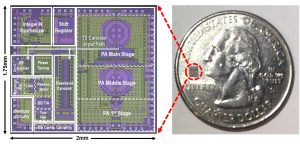
Figure 3: Chip Photo of the TSMC 40nm 6L-Metal Prototype Full Duplex Radio Front-end.
The ISSCC paper describes the high-frequency full duplex radio front-end integrated in an advanced nanometer-length (40nm) Taiwan Semiconductor Manufacturing Company (TSMC) process. The entire full duplex front-end chip (as shown in Figure 3) occupies a die area of less than 4mm2 and includes two self-interference cancellation paths, a frequency synthesizer, analog receiver and a noise canceling power amplifier.
“The Full Duplex Communication transceiver architecture contains a dual-path self-interference cancellation technique, which achieves the state-of-the-art performance,” Zhang said. “The research demonstrates a full radio front-end by complementing the experimental chip with an FPGA to emulate the baseband processor [Figure 4]. The overall embedded radio system self-calibrates the analog dual-path cancellation frontend chip. The configuration works together to develop an efficient method for wireless communication to address future demands for higher data rate”
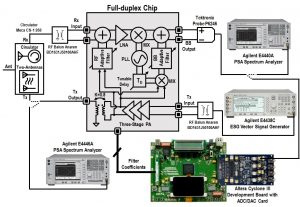
Figure 4: Measurement Setup of the entire Full Duplex Radio System, which includes the prototype chip and digital baseband emulator (FPGA).
Similar signal processing challenges exist in biological interfaces. At present, the PI and authors of this paper are involved with the Center for Sensorimotor Neural Engineering (CSNE) at the University of Washington, where they are exploring the use of similar full duplex techniques to conquer a longstanding problem with neural interfaces, mainly, the suppression of unwanted stimulation artifacts in the sense (recording) electronics.
Additional co-authors on the paper are electrical engineering graduate students Ali Najafi and Chenxin Su. This research was funded by the National Science Foundation, Google, Qualcomm, Marvell Technology Group and the Center for Design of Analog-Digital Integrated Circuits (CDADIC).